The thorny thought experiment has been turned into a real experiment — one that physicists use to probe the physics of information.
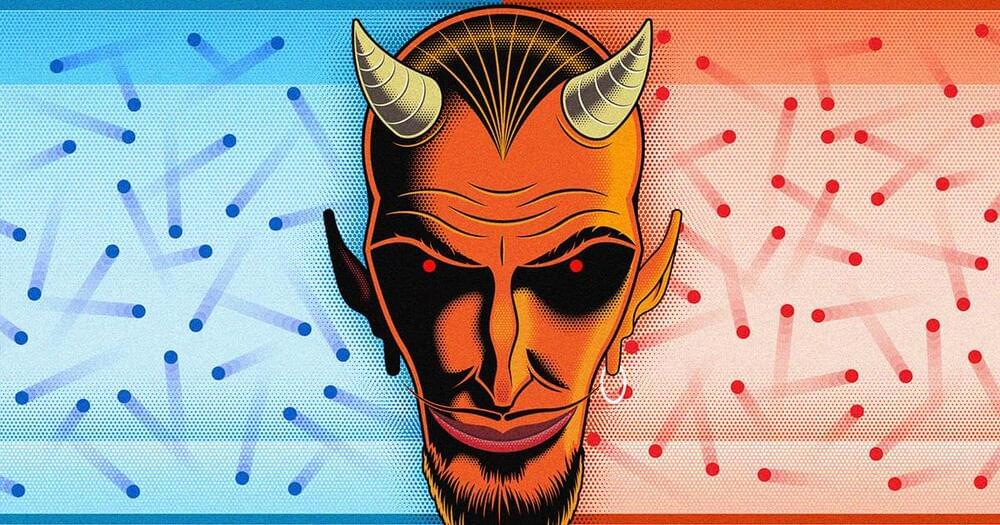
https://youtube.com/watch?v=V8cPdjO3a_U&feature=share
Find out what the world will be like a million years from now, as well as what kind of technology we’ll have available.
► All-New Echo Dot (5th Generation) | Smart Speaker with Clock and Alexa | Cloud Blue: https://amzn.to/3ISUX1u.
► Brilliant: Interactive Science And Math Learning: https://bit.ly/JasperAITechUniNet.
Timestamps:
0:00 No Physical Bodies.
1:51 Wormhole Creation.
2:44 Travel At Speed Of Light.
3:21 Type 3 Civilization.
4:52 Gravitational Waves.
5:46 Computers the Size of Planets.
6:56 Computronium.
I explain the following ideas on this channel:
* Technology trends, both current and anticipated.
* Popular business technology.
* The Impact of Artificial Intelligence.
* Innovation In Space and New Scientific Discoveries.
* Entrepreneurial and Business Innovation.
Subscribe link.
https://www.youtube.com/channel/UCpaciBakZZlS3mbn9bHqTEw.
Disclaimer:
Some of the links contained in this description are affiliate links.
As an Amazon Associate, I get commissions on orders that qualify.
This video describes the world and its technologies in a million years. Future technology, future technologies, tech universe, computronium, the world in a million years, digital immortality, wormhole, wormholes, faster than light, type 3 civilization, control gravity, planet sized computer, black hole energy extraction, black hole, black hole energy, and so on.
Dr. Ben Goertzel.
SingularityNET
The Coming Consciousness Explosion.
Bio: Dr. Ben Goertzel is a cross-disciplinary scientist, entrepreneur and author. Born in Brazil to American parents, in 2020 after a long stretch living in Hong Kong he relocated his primary base of operations to the rural Seattle area. He leads the SingularityNET Foundation, the OpenCog Foundation, and the AGI Society which runs the annual Artificial General Intelligence conference.
Dr. Goertzel also chairs the futurist nonprofit Humanity+, and serves as Chief Scientist of AI firms Singularity Studio, Rejuve, SingularityDAO and Xccelerando Media, all parts of the SingularityNET ecosystem. As Chief Scientist of robotics firm Hanson Robotics, he led the software team behind the Sophia robot; as Chief AI Scientist of Awakening Health he leads the team crafting the mind behind Sophia’s little sister Grace.
Dr. Goertzel’s research work encompasses multiple areas including artificial general intelligence, natural language processing, cognitive science, machine learning, computational finance, bioinformatics, virtual worlds, gaming, parapsychology, theoretical physics and more. He has published 25+ scientific books, ~150 technical papers, and numerous journalistic articles, and given talks at a vast number of events of all sorts around the globe.
Before entering the software industry Dr. Goertzel obtained his PhD in mathematics from Temple University in 1989, and served as a university faculty in several departments of mathematics, computer science and cognitive science, in the US, Australia and New Zealand.
After crunching a mountain of astronomy data, Clarissa Pavao, an undergraduate at Embry-Riddle Aeronautical University’s Prescott, Arizona campus, submitted her preliminary analysis. Her mentor’s response was swift and in all-caps: “THERE’S AN ORBIT!” he wrote.
That was when Pavao, a senior space physics major, realized she was about to become a part of something big—a paper in the journal Nature that describes a rare binary star system with uncommon features.
The paper, published on Feb. 1, 2023, and co-authored with Dr. Noel D. Richardson, assistant professor of Physics and Astronomy at Embry-Riddle, describes a twin-star system that is luminous with X-rays and high in mass. Featuring a weirdly circular orbit—an oddity among binaries—the twin system seems to have formed when an exploding star or supernova fizzled out without the usual bang, similar to a dud firecracker.
With simulations that go into finer details than ever before, Brooke Polak of the University of Heidelberg and Hubert Klahr at the Max Planck Institute for Astronomy (MPIA) have modeled a key phase in the formation of planets in our solar system: the way that centimeter-size pebbles aggregate into so-called planetesimals tens to hundreds kilometers in size. The simulation reproduces the initial size distribution of planetesimals, which can be checked against observations of present-day asteroids. It also predicts the prevalence of close binary planetesimals in our solar system.
In a new study published on arXiv and accepted for publication in The Astrophysical Journal, astrophysicists Brooke Polak from the University of Heidelberg and Hubert Klahr from the Max Planck Institute for Astronomy used simulations to derive key properties of so-called planetesimals—the intermediate-size bodies from which planets formed in our solar system roughly 4.5 billion years ago.
Using an innovative method for simulating planetesimal formation, the two researchers were able to predict the initial size distribution of planetesimals in our solar system: how many are likely to have formed in the different “size brackets” between roughly 10 km and 200 km.
❤️ Check out Weights & Biases and sign up for a free demo here: https://wandb.com/papers.
📝 The paper “Co-Writing Screenplays and Theatre Scripts with Language Models: An Evaluation by Industry Professionals” is available here:
https://deepmind.github.io/dramatron/details.html.
My latest paper on simulations that look almost like reality is available for free here:
https://rdcu.be/cWPfD
Or this is the orig. Nature Physics link with clickable citations:
https://www.nature.com/articles/s41567-022-01788-5
🙏 We would like to thank our generous Patreon supporters who make Two Minute Papers possible:
Aleksandr Mashrabov, Alex Balfanz, Alex Haro, Andrew Melnychuk, Benji Rabhan, Bryan Learn, B Shang, Christian Ahlin, Edward Unthank, Eric Martel, Geronimo Moralez, Gordon Child, Jace O’Brien, Jack Lukic, John Le, Jonas, Jonathan, Kenneth Davis, Klaus Busse, Kyle Davis, Lorin Atzberger, Lukas Biewald, Matthew Allen Fisher, Matthew Valle, Michael Albrecht, Michael Tedder, Nevin Spoljaric, Nikhil Velpanur, Owen Campbell-Moore, Owen Skarpness, Rajarshi Nigam, Ramsey Elbasheer, Richard Sundvall, Steef, Taras Bobrovytsky, Ted Johnson, Thomas Krcmar, Timothy Sum Hon Mun, Torsten Reil, Tybie Fitzhugh, Ueli Gallizzi.
If you wish to appear here or pick up other perks, click here: https://www.patreon.com/TwoMinutePapers.
Thumbnail background image credit: https://pixabay.com/images/id-1668918/
There are two aspects to a computer’s power: the number of operations its hardware can execute per second and the efficiency of the algorithms it runs. The hardware speed is limited by the laws of physics. Algorithms—basically sets of instructions —are written by humans and translated into a sequence of operations that computer hardware can execute. Even if a computer’s speed could reach the physical limit, computational hurdles remain due to the limits of algorithms.
These hurdles include problems that are impossible for computers to solve and problems that are theoretically solvable but in practice are beyond the capabilities of even the most powerful versions of today’s computers imaginable. Mathematicians and computer scientists attempt to determine whether a problem is solvable by trying them out on an imaginary machine.