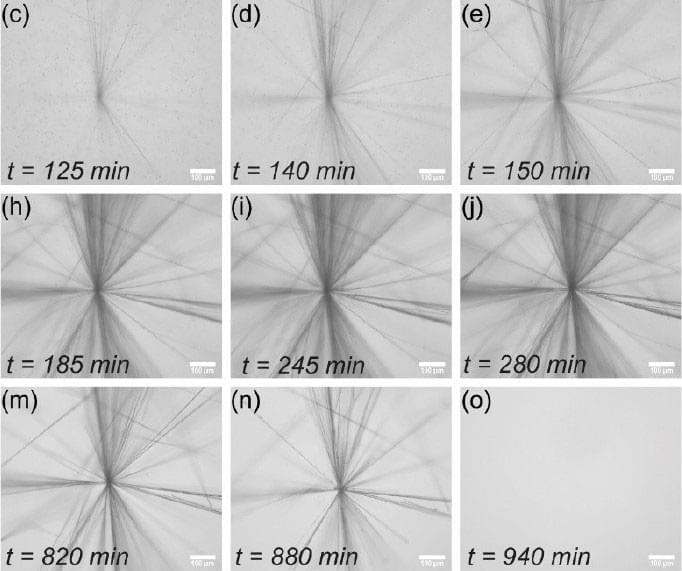
Self-assembled molecules are responsible for important cellular processes. Self-assembled structures such as microtubules or actin filaments are key to cell motility: change of shape, division or extension of membranes. These self-assembled entities have the peculiarity of being formed temporarily, since they require energy consumption. Inspired by nature, there is currently an active area of research that attempts to replicate this process of self-assembly artificially, using the so-called chemical reaction networks.
The control of self-assembly by means of chemical reaction networks is based on the activation of a monomer prone to self-assembly, which is then deactivated. In this way, the self-assembled structure requires a continuous energy consumption to perpetuate itself. From a chemical point of view, this energy is provided by a “fuel”, a chemical reagent. Depending on the availability of that energy source, the self-assembly process occurs or not.
Traditionally, highly reactive fuels have been used to carry out the activation, with little control over the deactivation process. This also implies that the activation and deactivation fuels tend to react with each other, making artificial dissipative self-assembly processes ineffective. In nature, these two processes are controlled by catalysts, which increases their efficiency. Thus, the introduction of catalysts in these processes and the control of their activity by external stimuli such as light are highly desirable, since they can limit part of these problems.