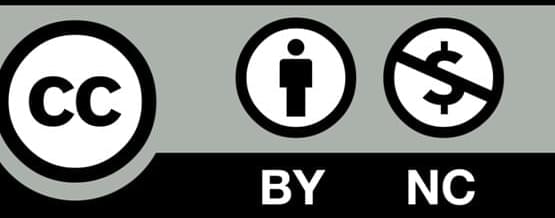
The Video Assistant Referees (VAR) was made official by the FIFA for the World Cup in Russia in order to end the refereeing controversies. this system is considered a total justice for football, VAR and the anti-dopping system have an Achilles’heel: The mind control with brain nanobots. Mind control is a reductive process in which a man is reduced to an animal, machine or slave. Nowadays, the mind control could be developed with invasive neurotechnology as brain nanobots that can control directly the activity of victim neurons stimulating or inhibiting them and thus, control different body’s functions like the motor functions. It could be used by nanomafias in sports like soccer and could being applied on football players of the teams that are participating, nowadays, in the current world cup. The FIFA should be prepared to avoid the mental control and the illicit use of brain nanobots, as they are regarding drugs using the anti-dopping in order to get the justice in world football.
Keywords: nanotechnology, brain, internet, interface brain-machine, crime, soccer.
The 2018 FIFA World Cup is the 21st FIFA World Cup, a quadrennial international football that is organized by FIFA. It is currently ongoing in Russia starting from 14 June and will end with the final match on 15 July 2018. The Video Assistant Referees (VAR) was made official by the FIFA for the World Cup in Russia in order to end the refereeing controversies. The FIFA implemented the VAR that is a live support system for referees, which gives them the option of changing decisions that could influence in the scoreboard or in the match incidences although this system is considered the final point for the controversies in refereeing decisions and represent for FIFA and most analysts, a total justice for football.1 The VAR and the anti-dopping system have an Achilles’ heel.