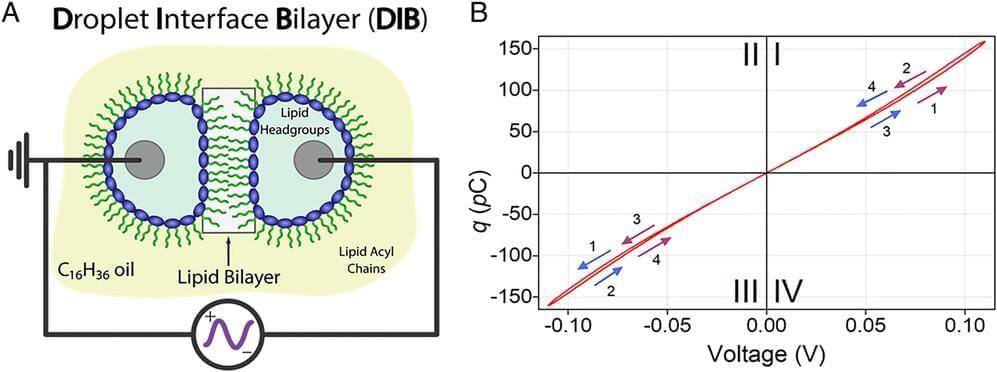
Ralph Lydic, professor in the UT Department of Psychology, and Dmitry Bolmatov, a research assistant professor in the UT Department of Physics and Astronomy, are part of a UT/ORNL research team studying how bio-inspired materials might inform the design of next-generation computers. Their results, published recently in the Proceedings of the National Academy of Sciences, could have big implications for both edge computing and human health.
Scientists at ORNL and UT discovered an artificial cell membrane is capable of long-term potentiation, or LTP, a hallmark of biological learning and memory. This is the first evidence that a cell membrane alone—without proteins or other biomolecules embedded within it—is capable of LTP that persists for many hours. It is also the first identified nanoscale structure in which memory can be encoded.
“When facilities were shut down as a result of COVID, this led us to pivot away from our usual membrane research,” said John Katsaras, a biophysicist in ORNL’s Neutron Sciences Directorate specializing in neutron scattering and the study of biological membranes at ORNL. “Together with postdoc Haden Scott, we decided to revisit a system previously studied by Pat Collier and co-workers, this time with an entirely different electrical stimulation protocol that we termed ‘training.’”.