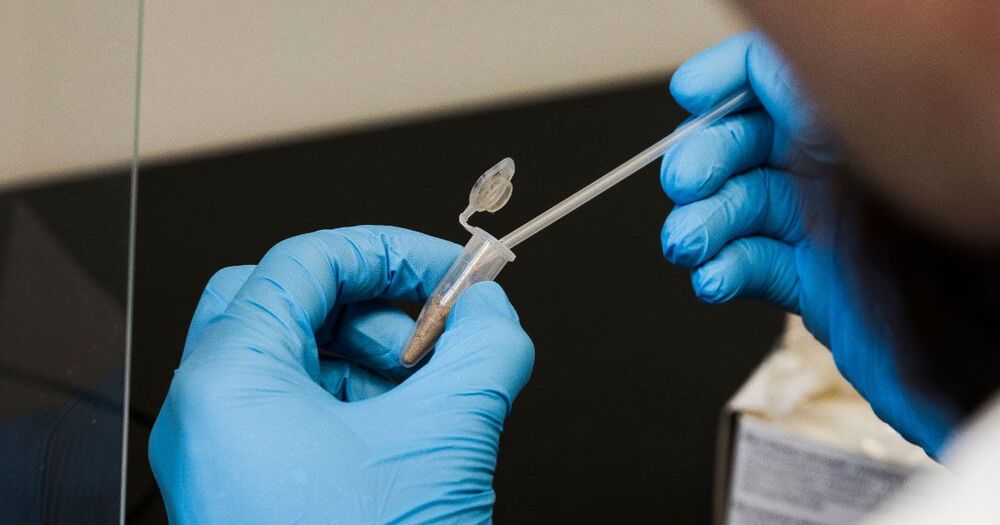
In March 2017, Read and his Penn State colleague David Kennedy published a paper in the Proceedings of the Royal Society B in which they outlined several strategies that vaccine developers could use to ensure that future vaccines don’t get punked by evolutionary forces. One overarching recommendation is that vaccines should induce immune responses against multiple targets. A number of successful, seemingly evolution-proof vaccines already work this way: After people get inoculated with a tetanus shot, for example, their blood contains 100 types of unique antibodies, all of which fight the bacteria in different ways. In such a situation, it becomes much harder for a pathogen to accumulate all the changes needed to survive. It also helps if vaccines target all the known subpopulations of a particular pathogen, not just the most common or dangerous ones. Richard Malley and other researchers at Boston Children’s Hospital are, for instance, trying to develop a universal pneumococcal vaccine that is not serotype-specific.
Vaccines should also bar pathogens from replicating and transmitting inside inoculated hosts. One of the reasons that vaccine resistance is less of a problem than antibiotic resistance, Read and Kennedy posit, is that antibiotics tend to be given after an infection has already taken hold — when the pathogen population inside the host is already large and genetically diverse and might include mutants that can resist the drug’s effects. Most vaccines, on the other hand, are administered before infection and limit replication, which minimizes evolutionary opportunities.
But the most crucial need right now is for vaccine scientists to recognize the relevance of evolutionary biology to their field. Last month, when more than 1000 vaccine scientists gathered in Washington, D.C., at the World Vaccine Congress, the issue of vaccine-induced evolution was not the focus of any scientific sessions. Part of the problem, Read says, is that researchers are afraid: They’re nervous to talk about and call attention to potential evolutionary effects because they fear that doing so might fuel more fear and distrust of vaccines by the public — even though the goal is, of course, to ensure long-term vaccine success. Still, he and Kennedy feel researchers are starting to recognize the need to include evolution in the conversation. “I think the scientific community is becoming increasingly aware that vaccine resistance is a real risk,” Kennedy said.