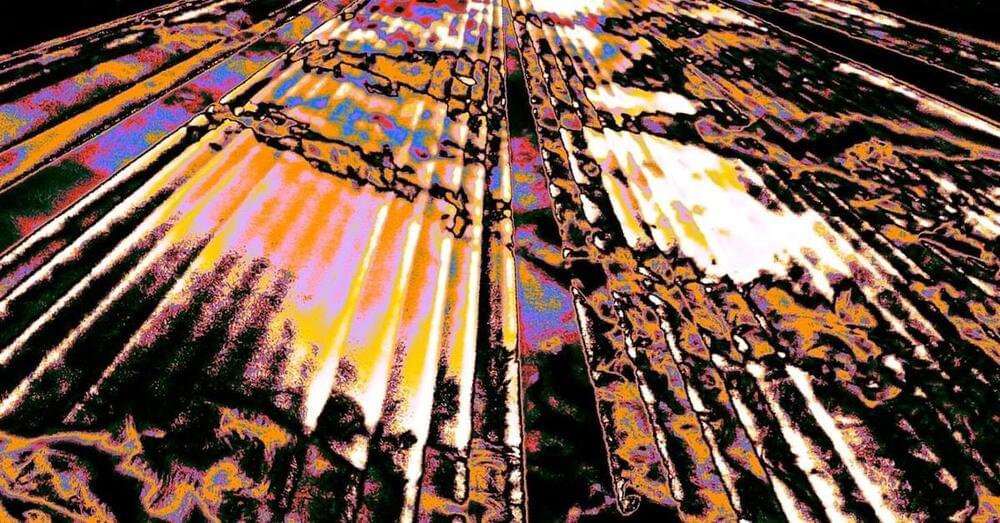
At least, that was the assumption in the second half of the 19th century. This scenario became known as the “heat death” of the universe, and it seemed to be the nail in the coffin for any optimistic cosmology that promised, or even allowed, eternal life and consciousness. For example, one of the most popular cosmological models of the time was put forth by the evolutionary theorist Herbert Spencer, a contemporary of Charles Darwin who was actually more famous than him during their time. Spencer believed that the flow of energy through the universe was organizing it. He argued that biological evolution was just part of a larger process of cosmic evolution, and that life and human civilization were the current products of a process of continual cosmic complexification, which would ultimately lead to a state of maximal complexity, integration and balance among all things.
When the prominent Irish physicist John Tyndall told Spencer about the heat death hypothesis in a letter in 1858,” Spencer wrote him back to say it left him “staggered”: “Indeed, not seeing my way out of the conclusion, I remember being out of spirits for some days afterwards. I still feel unsettled about the matter.”
Things got even gloomier when the Austrian physicist Ludwig Boltzmann put forward a new statistical interpretation of the second law in the latter half of the 19th century. That was when the idea that the universe is growing more disordered came into the picture. Boltzmann took the classical version of the second law — that useful energy inevitably dissipates — and tried to give it a statistical explanation on the level of molecules colliding and spreading out. He used one of the simplest models possible: a gas confined to a box.