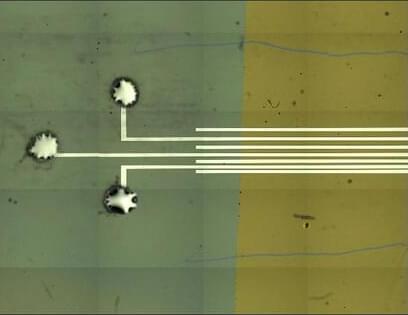
To achieve high intrinsic gain (Ai) in OTFTs, it is necessary to enlarge output resistance (ro) or transconductance (gm) according to a typical formula of Ai = gmro, which is very difficult for conventional OTFTs because of inherent device structure and operating mode limitations (11, 12). Recently, the “Schottky barrier” (SB) strategy based on metal-semiconductor junction (MS junction) has been adopted in TFTs to pursue high-gain and low-saturation voltage, including subthreshold SB-TFTs (11, 12, 15, 16) and source-gated transistors (17, 18). Unfortunately, the subthreshold transistors are limited in low and narrow subthreshold operating region rather than the normal ON-state region (namely, the normal voltage operating region in a typical TFT), which are difficult to be compatible with typical circuits. As far as we know, the ultrahigh-gain (1000) OTFTs operating in the ON-state region have not been previously reported. On the other hand, the state-of-the-art OTFTs above have mostly suffered from uncontrollable barriers owing to energy-level mismatches and a series of complex interface problems, such as Fermi-level pinning and interface chemical disorder (19). In this case, considerable low-energy carriers are allowed to pass through the junction by thermionic field emission and tunneling models instead of thermionic emission model, which is not conducive to obtaining a high output resistance and high intrinsic gain. Most barrier heights in MS junction do not conform to the prediction value of Schottky-Mott rule. Theoretically, an ideal and high-quality barrier with thermionic emission model allows the rapid depletion of carriers at the source electrode, thus yielding ultrahigh gain, infinite output resistance, and low saturation voltage (11, 12). In addition, infinite output resistance at the saturation regime indicates that the output current is very stable and flat. This performance is helpful because only a single OTFT is used as a simplified current stabilizer in circuits without complex circuit design, which benefits low power and low cost in circuits. Therefore, it is necessary to develop a high-quality barrier strategy to modulate charge injection to meet the requirements of ultrahigh-gain OTFTs.
Here, we demonstrate a metal-barrier interlayer-semiconductor (MBIS) junction to prepare high-performance MBIS-OTFT with an ultrahigh gain of ~104 in the ON-state region, low saturation voltage, almost negligible hysteresis, and good stability. On the basis of low-energy processes and in situ surface oxidation technology, the high-quality van der Waals MBIS junction with wide-bandgap semiconductor (mainly Ga2O3) interlayer is achieved, allowing for an adjustable barrier height and thermionic emission properties. A series of in situ experiments and simulations revealed the relationship between the barriers and the device’s performance. Furthermore, as demonstrations, a simplified current stabilizer and an ultrahigh-gain organic inverter are exhibited without complex circuit design.